NASA’s revolutionary new space observatory, the James Webb Space Telescope, successfully launched into space after taking off on top of a European Ariane 5 rocket from South America on December 25, 2021. The launch marks the beginning of one of the most anticipated NASA missions in decades, a program that promises to transform how we study the deepest depths of the Universe.
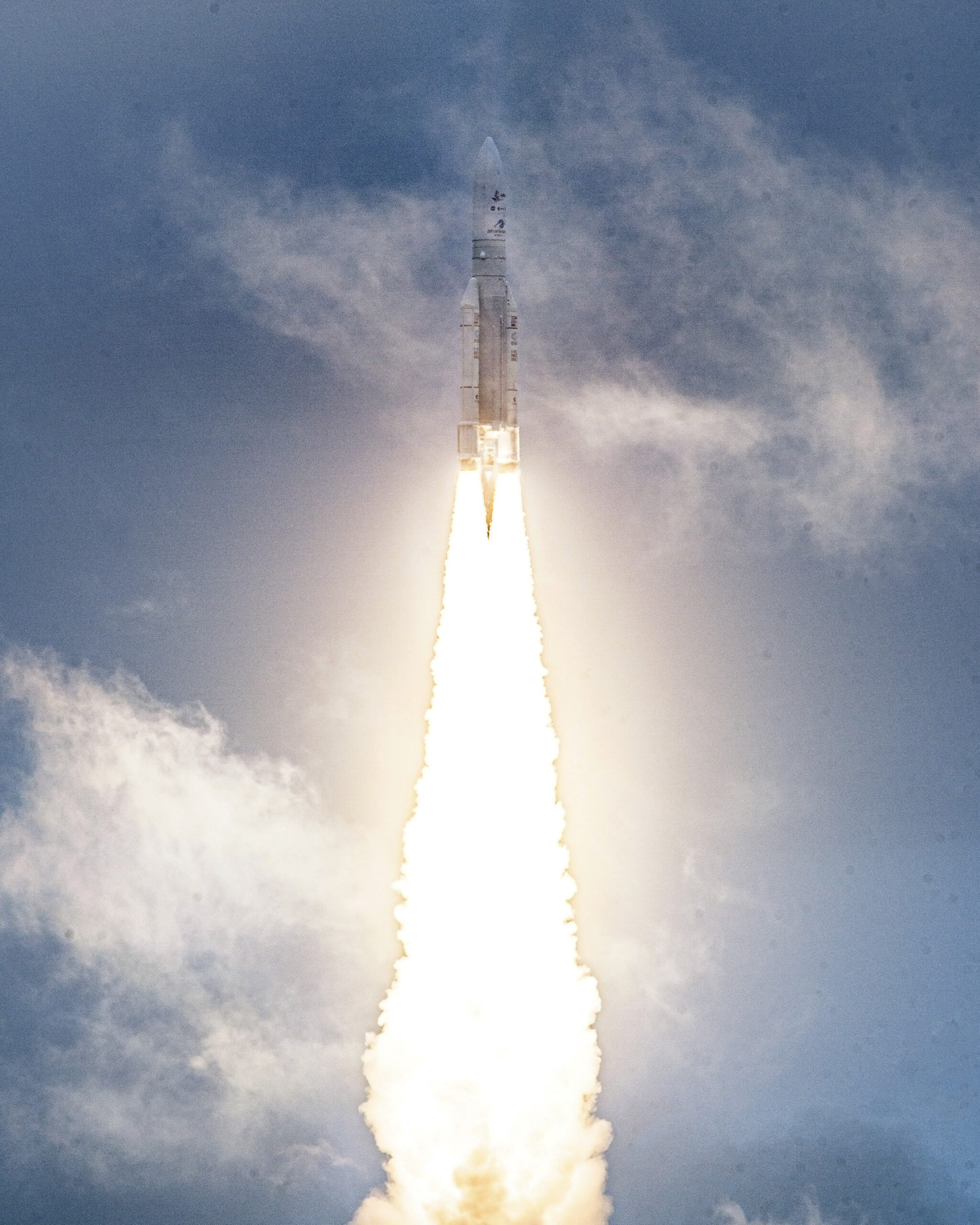
Arianespace’s Ariane 5 rocket launches with NASA’s James Webb Space Telescope onboard, Saturday, Dec. 25, 2021, from the ELA-3 Launch Zone of Europe’s Spaceport at the Guiana Space Centre in Kourou, French Guiana. The James Webb Space Telescope (sometimes called JWST or Webb) is a large infrared telescope with a 21.3 foot (6.5 meter) primary mirror. The observatory will study every phase of cosmic history—from within our solar system to the most distant observable galaxies in the early universe. Photo Credit: (NASA/Chris Gunn)
JWST is often considered the successor to the Hubble Space Telescope, which has been orbiting the Earth since 1990. However, the James Webb Space Telescope is designed to conduct an array of scientific experiments, such as using its sophisticated infrared imaging technology to look farther back in time than its predecessor. JWST’s mirror will dwarf Hubble’s mirror, which is just 8 feet, or 2.4 meters, wide. This next-generation observatory promises to be 10 to 100 times more sensitive than Hubble and much more capable of picking up distant, faint objects. To emphasize JWST’s impressive capabilities, NASA claims that the telescope would be able to pick up the infrared light emitted by a bumblebee located at the distance of the Moon.
Astronomers have dreamt of JWST’s capabilities for the last two and a half decades, but turning those dreams into reality has been a grueling process. Scientists formally proposed a massive infrared space telescope in 1996, and early mission engineers hoped to launch such an observatory as early as 2007 for a price tag as low as $1 billion. However, creating the spacecraft took much more time and money than anyone anticipated. JWST missed one target launch date after the next, while its budget ballooned by billions of dollars. Congress even tried to cancel the project at one point over the rising costs but agreed to continue funding the mission if NASA stuck to a cost cap of $8.8 billion. However, NASA blew through that, and the entire mission cost now sits at $9.7 billion.
But the telescope’s work is just beginning. JWST had to fly to space folded up, as it’s too massive to fit on any existing rocket in its final form. NASA and Europe agreed to fly JWST on Arianespace’s premiere Ariane 5 rocket back in the early 2000s, citing the vehicle’s reliability. Although Ariane 5 is nearly 18 feet, or 5.4 meters, wide, it was still not big enough to carry JWST to space completely unfurled.
Now a long to-do list of deployments is in store for the telescope. JWST already deployed its solar array in order to get power from the Sun, and tomorrow, it will deploy its high gain antenna to communicate with Earth. After that, the telescope will slowly deploy various beams and structures, reconfiguring its shape so that it can observe infrared light of the distant Universe. Perhaps its most crucial deployment is its sunshield, which consists of five thin layers of a material called Kapton. In order to see in infrared light, JWST must stay incredibly cold. The sunshield is key for absorbing heat from our Sun, allowing JWST to remain a frigid -370 degrees Fahrenheit. The shield’s deployment must be perfect to guarantee the mission’s success.
NASA led JWST’s development in collaboration with ESA and the Canadian Space Agency (CSA). The NASA Goddard Space Flight Center (GSFC) in Maryland managed telescope development, the Space Telescope Science Institute in Baltimore operates JWST, and the prime contractor was Northrop Grumman. The telescope is named after James E. Webb, who was the administrator of NASA from 1961 to 1968 during the Mercury, Gemini, and Apollo programs.
JWST’s primary mirror consists of 18 hexagonal mirror segments made of gold-plated beryllium which combined create a 6.5-meter (21 ft) diameter mirror, compared with Hubble’s 2.4 m (7.9 ft). This gives the Webb telescope a light-collecting area of about 25 square meters, about 6 times that of Hubble. Unlike Hubble, which observes in the near ultraviolet, visible, and near infrared (0.1–1.7 μm) spectra, JWST will observe in a lower frequency range, from long-wavelength visible light (red) through mid-infrared (0.6–28.3 μm). The telescope must be kept extremely cold, below 50 K (−223 °C; −370 °F), to observe faint signals in the infrared without interference from other sources of warmth. It is deployed in a solar orbit near the Sun–Earth L2 Lagrange point, about 1.5 million kilometers (930,000 mi) from Earth, where its five layer kite-shaped sunshield protects it from warming by the Sun, Earth, and Moon.
Development began in 1996 for a launch initially planned for 2007 with a US$500 million budget. There were many delays and cost overruns, including a major redesign in 2005, a ripped sunshield during a practice deployment, recommendations from an independent review board, a threat by the U.S. Congress to cancel the project, the COVID-19 pandemic, and problems with the telescope itself. The high-stakes nature of the launch and the telescope’s complexity were remarked upon by the media, scientists and engineers. Construction was completed in late 2016, followed by years of extensive testing before launch. The total project cost is now expected to be about US$9.7 billion.
Features
The James Webb Space Telescope has a mass that is about half of Hubble Space Telescope’s mass. The JWST has a 6.5-meter (21 ft)-diameter gold-coated beryllium primary mirror made up of 18 separate hexagonal mirrors. The mirror has a polished area of 26.3 m2 (283 sq ft), of which 0.9 m2 (9.7 sq ft) is obscured by the secondary support struts,[8] giving a total collecting area of 25.4 m2 (273 sq ft). This is over six times larger than the collecting area of Hubble’s 2.4-meter (7.9 ft) diameter mirror, which has a collecting area of 4.0 m2 (43 sq ft). The mirror has a gold coating to provide infrared reflectivity and durability.
JWST is designed primarily for near-infrared astronomy, but can also see orange and red visible light, as well as the mid-infrared region, depending on the instrument. It can detect objects up to 100 times fainter than Hubble can, and objects much earlier in the history of the universe, back to redshift z≈20 (about 180 million years cosmic time after the Big Bang). For comparison, the earliest stars are thought to have formed between z≈30 and z≈20 (100-180 million years cosmic time), and the first galaxies may have formed around redshift z≈15 (about 270 million years cosmic time). Hubble is unable to see further back than very early reionization at about z≈11.1 (galaxy GN-z11, 400 million years cosmic time).
The design emphasizes the near to mid-infrared for three main reasons:
- high-redshift (very early and distant) objects have their visible emissions shifted into the infrared, and therefore their light can only be observed today via infrared astronomy;
- colder objects such as debris disks and planets emit most strongly in the infrared;
- these infrared bands are difficult to study from the ground or by existing space telescopes such as Hubble.
Ground-based telescopes must look through Earth’s atmosphere, which is opaque in many infrared bands (see figure of atmospheric absorption). Even where the atmosphere is transparent, many of the target chemical compounds, such as water, carbon dioxide, and methane, also exist in the Earth’s atmosphere, vastly complicating analysis. Existing space telescopes such as Hubble cannot study these bands since their mirrors are insufficiently cool (the Hubble mirror is maintained at about 15 °C (288 K; 59 °F)) thus the telescope itself radiates strongly in the infrared bands.
JWST can also observe nearby objects, including objects in the Solar System, having an apparent angular rate of motion of 0.030 arc seconds per second or less. This includes all planets and satellites, comets, and asteroids beyond Earth’s orbit, and “virtually all” known Kuiper Belt Objects.[10] In addition, it can observe opportunistic and unplanned targets within 48 hours of a decision to do so, such as supernovae and gamma ray bursts.
Location and orbit
JWST operates in a halo orbit, circling around a point in space known as the Sun-Earth L2 Lagrange point, approximately 1,500,000 km (930,000 mi) beyond Earth’s orbit around the Sun. Its actual position varies between about 250,000 km (160,000 mi) and 832,000 km (517,000 mi) from L2 as it orbits, keeping it out of both Earth and Moon’s shadow. By way of comparison, Hubble orbits 550 km (340 mi) above Earth’s surface, and the Moon is roughly 400,000 km (250,000 mi) from Earth.
Objects near this Sun-Earth L2 point can orbit the Sun in synchrony with the Earth, allowing the telescope to remain at a roughly constant distance with continuous orientation of its unique sunshield and equipment bus toward the Sun, Earth and Moon. Combined with its wide shadow-avoiding orbit, the telescope can simultaneously block incoming heat and light from all three of these bodies and avoid even the smallest changes of temperature from Earth and Moon shadows that would affect the structure, yet still maintain uninterrupted solar power and Earth communications on its sun-facing side. This arrangement keeps the temperature of the spacecraft constant and below the 50 K (−223 °C; −370 °F) necessary for faint infrared observations.
Scientific instruments
The Integrated Science Instrument Module (ISIM) is a framework that provides electrical power, computing resources, cooling capability as well as structural stability to the Webb telescope. It is made with bonded graphite-epoxy composite attached to the underside of Webb’s telescope structure. The ISIM holds the four science instruments and a guide camera.
- NIRCam (Near InfraRed Camera) is an infrared imager which will have a spectral coverage ranging from the edge of the visible (0.6 μm) through to the near infrared (5 μm).There are 10 sensors each of 4 megapixels. NIRCam will also serve as the observatory’s wavefront sensor, which is required for wavefront sensing and control activities, used to align and focus the main mirror segments. NIRCam was built by a team led by the University of Arizona, with principal investigator Marcia J. Rieke. The industrial partner is Lockheed-Martin’s Advanced Technology Center in Palo Alto, California.
- NIRSpec (Near InfraRed Spectrograph) will also perform spectroscopy over the same wavelength range. It was built by the European Space Agency at ESTEC in Noordwijk, Netherlands. The leading development team includes members from Airbus Defence and Space, Ottobrunn and Friedrichshafen, Germany, and the Goddard Space Flight Center; with Pierre Ferruit (École normale supérieure de Lyon) as NIRSpec project scientist. The NIRSpec design provides three observing modes: a low-resolution mode using a prism, an R~1000 multi-object mode, and an R~2700 integral field unit or long-slit spectroscopy mode.Switching of the modes is done by operating a wavelength preselection mechanism called the Filter Wheel Assembly, and selecting a corresponding dispersive element (prism or grating) using the Grating Wheel Assembly mechanism. Both mechanisms are based on the successful ISOPHOT wheel mechanisms of the Infrared Space Observatory. The multi-object mode relies on a complex micro-shutter mechanism to allow for simultaneous observations of hundreds of individual objects anywhere in NIRSpec’s field of view. There are two sensors each of 4 megapixels. The mechanisms and their optical elements were designed, integrated and tested by Carl Zeiss Optronics GmbH (today Hensoldt) of Oberkochen, Germany, under contract from Astrium.
- MIRI (Mid-InfraRed Instrument) will measure the mid-to-long-infrared wavelength range from 5 to 27 μm. It contains both a mid-infrared camera and an imaging spectrometer.MIRI was developed as a collaboration between NASA and a consortium of European countries, and is led by George Rieke (University of Arizona) and Gillian Wright (UK Astronomy Technology Centre, Edinburgh, Scotland, part of the Science and Technology Facilities Council (STFC)).MIRI features similar wheel mechanisms to NIRSpec which are also developed and built by Carl Zeiss Optronics GmbH (today Hensoldt) under contract from the Max Planck Institute for Astronomy, Heidelberg, Germany. The completed Optical Bench Assembly of MIRI was delivered to Goddard Space Flight Center in mid-2012 for eventual integration into the ISIM. The temperature of the MIRI must not exceed 6 K (−267 °C; −449 °F): a helium gas mechanical cooler sited on the warm side of the environmental shield provides this cooling.
- FGS/NIRISS (Fine Guidance Sensor and Near Infrared Imager and Slitless Spectrograph), led by the Canadian Space Agency under project scientist John Hutchings (Herzberg Astronomy and Astrophysics Research Centre, National Research Council), is used to stabilize the line-of-sight of the observatory during science observations. Measurements by the FGS are used both to control the overall orientation of the spacecraft and to drive the fine steering mirror for image stabilization. The Canadian Space Agency is also providing a Near Infrared Imager and Slitless Spectrograph (NIRISS) module for astronomical imaging and spectroscopy in the 0.8 to 5 μm wavelength range, led by principal investigator René Doyon at the Université de Montréal. Because the NIRISS is physically mounted together with the FGS, they are often referred to as a single unit; however, they serve entirely different purposes, with one being a scientific instrument and the other being a part of the observatory’s support infrastructure.
NIRCam and MIRI feature starlight-blocking coronagraphs for observation of faint targets such as extrasolar planets and circumstellar disks very close to bright stars.
The infrared detectors for the NIRCam, NIRSpec, FGS, and NIRISS modules are being provided by Teledyne Imaging Sensors (formerly Rockwell Scientific Company). The James Webb Space Telescope (JWST) Integrated Science Instrument Module (ISIM) and Command and Data Handling (ICDH) engineering team uses SpaceWire to send data between the science instruments and the data-handling equipment.
It’s really a great historical moment.